The small-satellite market is booming but smallsat launches face severe bottlenecks. Europe needs to develop and secure a microlauncher solution.
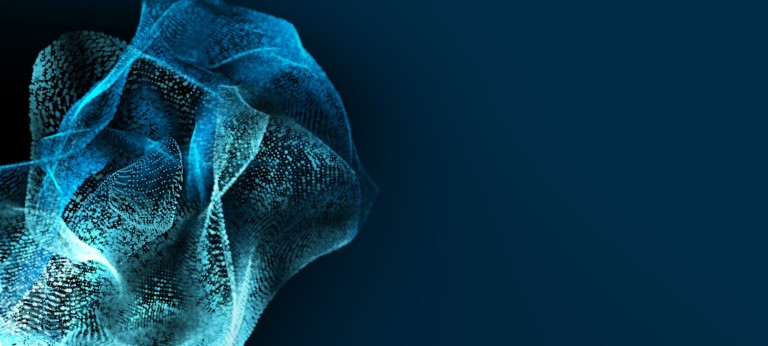
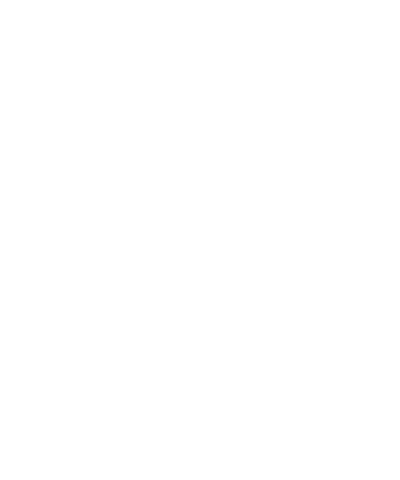
Space-based solar power
By Manfred Hader and Martin Hoyer
Helping decarbonize Europe and make it more energy resilient thanks to electricity generated in space
Exploring new green energy sources is now more urgent and relevant than ever. Global warming and independent, sovereign sourcing of green energy are becoming ever more pressing issues. In addition to the decarbonization challenge, the recent geopolitical crises have caused turmoil in the energy markets and a surge in electricity prices. It has become clear how vital energy resilience is for Europe. In this context, Roland Berger, in partnership with OHB System AG, has conducted a comprehensive cost-benefit study and impact analysis of a space-based solar power (SBSP) program conducted for the European Space Agency (ESA) between February and July 2022. This study aimed to disrupt the thinking about how we generate and transmit energy, to achieve carbon neutrality by 2050. To meet this challenge of our generation, we are convinced that further innovative solutions will be needed.
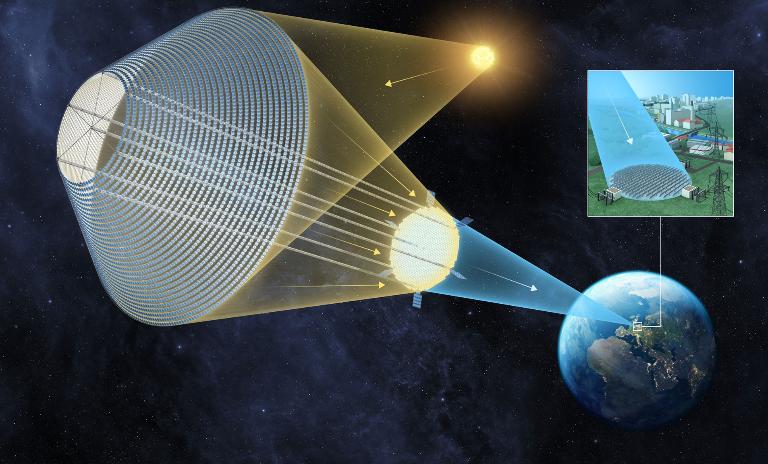
"Space-based solar power can contribute to the decarbonization of the European energy supply by providing high-density renewable energy to locations where it is needed the most."
What is Space-based solar power?
Space-based solar power (SBSP) is the concept of collecting solar power with a spacecraft in Earth orbit and distributing it to Earth. Unlike terrestrial systems, SBSP has the advantage of collecting solar energy in space, leading to a higher collection rate due to the lack of a diffusing atmosphere, and a longer collection period by placing a solar collector in an orbiting location.
In addition, there is no downtime due to the Earth's rotation (day/night cycle) and periods of eclipses are reduced or even avoided entirely. The system converts the sunlight to electricity, which is beamed to Earth wirelessly, most likely by radio frequency waves. As a green energy source, SBSP could provide green electricity to the grid 24/7, creating a renewable baseload capacity similar to that of nuclear power plants. An SBSP system is broken down into three main segments: the space segment, the ground segment and the launch segment. The space segment contains the platform in orbit, which is composed of elements such as the reflectors, the energy conversion and the wireless power transmission systems. The ground segment contains all elements needed to receive, convert and transmit the energy into the terrestrial grid. The launch segment comprises the launch systems to deliver the infrastructure into orbit, such as heavy reusable launchers or orbital transfer vehicles.
Technological feasibility
The basic technical feasibility of SBSP has already been confirmed by multiple studies since 2006. However, SBSP technologies are still in their very early stages of development. The relatively high upfront costs for the implementation, coupled with skepticism from decision-makers, have so far restricted their development. Although many of the building blocks for SBSP are based on existing or emerging technologies for space and onground applications, significant developments are still required to enable such a system. The development and construction of large modular structures in space is a major challenge. So far, the largest human-made structure in space is the International Space Station (ISS), with an overall envelope of around 8,000 m², as compared with an SBSP satellite, which can have an overall area of more than 15 km². In addition, low-cost, high-cadence heavy-lift, launchers are therefore necessary to enable SBSP. Even with the largest and most powerful launchers available today, such as Europe's Ariane 5 (and soon Ariane 6 in its 64 version), it would still require a very high launch cadence and a drastic reduction of launch costs to deliver into space the infrastructure necessary for a fully operational SBSP.
Cost competitiveness of SBSP vs. other technologies
Unsurprisingly, the main cost driver for the SBSP is the space segment. To achieve cost competitiveness, expected disruptions in critical technology fields are taken into account, which leads to lower costs. The competitiveness of SBSP vs. other clean energy sources was measured using the value-adjusted levelised cost of electricity (VALCoE) introduced by the International Energy Agency (IEA). The analysis also considered the high energy market uncertainty for electricity costs using four scenarios.
Based on the cost assumption of SBSP, the projected value-adjusted levelised cost could even reach 69 EUR/MWh for the first system. Applying a learning curve metric already shows cost reduction potential in the setup of the first 10 SPS station deployments, leading to an estimated VALCoE of 49 EUR/MWh. This means that SBSP has the potential to even become a cost-competitive renewable technology while also providing baseload generation capacity. Depending on the pace of climate target implementations and the state of energy supply, future electricity prices can range between 25 and 300 EUR/MWh.
"US and China have already joined the race for SBSP. To ensure sovereign energy supply, Europe needs its own SBSP capabilities."
How to make it happen
If launched, this project could have a considerable impact, especially considering that the energy sector is highly critical, affecting all the sectors of the economy. In the case of Europe, SBSP as part of the electricity mix could largely contribute to the decarbonization of the energy sector in the upcoming decades, with the potential to turn solar power into a renewable baseload capacity. We believe SBSP has the potential to contribute to the green energy transition and energy independence of Europe by providing high-density renewable and reliable energy to where it can have the most impact on decarbonization and sovereignty. To do so, it is key to demonstrate the technological and financial viability of SBSP. To address these challenges, Roland Berger recommends the following four successive steps:
- Step 1: Raise awareness (2022–2025):
Despite the theoretical potential of SBSP to provide a clean and sustainable source of energy, the system remains mainly unknown as compared with other long-term (and still under development) technologies such as nuclear fusion. Therefore, it is crucial to communicate the topic and its potential to different stakeholders and decision-makers in the public and private sectors, notably in the energy sector. - Step 2: Secure resources to mature the required technologies (2022–2030):
Several technologies still at low maturity will need to reach the technological readiness level necessary to prove to decision-makers the viability of SBSP. It is expected that some of the required technologies will gain maturity in the context of other development programs launched by public research agencies. On the other hand, other technologies (for example wireless power transmission) will require dedicated resources and should be prioritized. - Step 3: Launch a demonstrator to confirm the viability of SBSP (2024–2035):
A demonstrator program will be a structuring step to bring certainty and prove or refute the technical and financial feasibility of an SBSP. This step will allow the performance of the complete system architecture in the three segments (space, ground, launch) to be confirmed through a real-life design with industrial and financial risks that should still be relatively mitigated. - Step 4: Launch an ambitious and bankable program with public and private partners (2035 onward):
Finally, when the interests of the different involved parties are aligned and economic rationale and technical feasibility are proven, the launch of a large-scale program should be the next step. Mitigating risks associated with the deployment of such a program will be critical to supporting industrial activities (for example, by guaranteeing to purchase the electricity produced during the exploitation).
If you want to learn more about the results of our study, you can download the executive summary from the European Space Agency website here .
Register now to receive regular insights into Aerospace & Defense topics.